Soon after their plane touched down in Stockholm, George Smith and his wife of 38 years, Marjorie Sable, found themselves immersed in a cocoon of rock-star-like celebrity.
Ushered into a private airport lounge, they helped themselves to a small but bespoke buffet of coffee and snacks. Moments later, an attaché from the Swedish foreign service introduced himself, explaining that he was part of a team of helpers who would see to their personal needs, chauffeur them to and from events, and shield them, if they so desired, from the eager clutch of autograph seekers waiting outside their hotel. They arrived at that five-star hotel — the venerable Grand Hôtel — as part of a motorcade of gleaming black Volvo SUVs, each emblazoned in gold with the purpose of their visit: The Nobel Prize.
“It was incredible, really” Smith says. “Like a cross between Disneyland and Downton Abbey,” adds Sable.
The 2018 Nobel Prize in Chemistry was awarded to Smith, now a distinguished professor emeritus of biological sciences, along with two additional researchers, Caltech’s Frances Arnold and Sir Gregory Winter of Britain’s Medical Research Council Laboratory of Molecular Biology, on Dec. 10.
Arnold, an engineer, was honored with half the prize for her insights into how a “directed” form of natural selection could help create useful enzymes. Among her celebrated achievements has been the use of such molecules to create more environmentally friendly biofuels, agricultural chemicals, paper products and pharmaceuticals.
Winter, who shared the second half of the prize with Smith, was recognized for his use of bacteriophages, viruses that infect bacteria, to initiate a different form of human-induced Darwinism. From his lab in Cambridge, England, he and his team directed multiple generations of antibodies to evolve into promising therapeutic agents, among them the blockbuster rheumatoid arthritis drug Humira.
Neither of these achievements would have been possible without Smith. His creation of the now-renowned “phage display” not only laid the groundwork for both scientists’ breakthroughs but also for the rise of a vast, phage-related research enterprise now spanning the globe.
At a packed MU press conference following the prize announcement Oct. 10, Smith, a man for whom modesty comes naturally, downplayed his role. He said he knew his initial publications would be of interest to other molecular biologists. But he hadn’t a clue as to where they might lead.
“There is no way you could predict that this would be something really important,” Smith told the crowd. “Certainly, I didn’t. Nobody in my lab realized it was something, for example, that might lead to medicines.” Indeed, before their own star turn in Stockholm, phages have seldom received the red-carpet treatment. In the hours after the Nobel announcement, even the world’s most seasoned science journalists found themselves scrambling to make sense of them. But their ubiquity more than compensates for their pop culture anonymity.
Phages, in short, are the most common organism on Earth. A recent essay published by the National Institutes of Health estimated there are 1031 phage particles on the planet, “an impossibly large number that translates into approximately a trillion phages for every grain of sand in the world.” These numbers represent an extraordinarily diverse pool of genetic material, perhaps nature’s largest reservoir of unexplored genes and proteins.
Their diversity notwithstanding, individual phages are extraordinarily simple. Each consists of a single small chromosome encapsulated in a shell of “coat” proteins. First identified at the beginning of the 20th century, they have long intrigued the world’s scientists. Early work centered on identifying and deploying phages that could infect and kill pathogenic bacteria, research that was largely displaced by the development of antibiotics. Later scientists focused on developing phages as model organisms — their simplicity made them perfect for investigating processes such as genetic replication, transcription and protein synthesis that were difficult to observe in more complex creatures.
Like any biologist focused on the molecular level, Smith was well-acquainted with phages by the time he joined MU’s faculty in the summer of 1975. Smith had even worked with them as an undergraduate at Haverford College, where the academically gifted son of an Army officer enrolled in 1959.
By the mid-1970s, he was five years removed from a Harvard doctorate and had just completed a postdoctoral fellowship with the eminent immunologist Oliver Smithies at the University of Wisconsin. Both scientists approached their explorations in ways that, unlike previous “classical” methods, emphasized molecular-level research.
These ideas were just catching on at Mizzou, and the university was keen to engage the young scientist. Smith recalls showing up for his job interview with “a scruffy beard and a ponytail,” a look signaling his readiness to shake things up. He presented a long list of work-related “wants.” He got them all.
“I was one of the favored hires in the ‘new biology,’ as it was called in those days — what we now call cellular and molecular biology,” says Smith, whose beard is still prominent but now reasonably well-trimmed. “But I didn’t stay long in molecular immunology. Within a year and a half I had set my sights on another project — the first project that involved filamentous phages.”

Even future Nobel Prize winners advise and teach students. Smith, right, and Professor Emeritus Billy Cumbie work with incoming freshmen, circa 1994.
Smith is tall and slim. These days he carries himself with a slight stoop, as if four decades of looking down to engage less-lanky colleagues has left a permanent bow. When approached, his gaze is direct and welcoming. He laughs often and is skilled at gently deflecting reporters’ phage-related illiteracy, a holdover, undoubtedly, from years of describing his work to the molecular-biologically challenged.
As infectious agents, phages had long been useful to scientists needing vectors capable of transferring one organism’s genetic material to another. Smith’s new project aimed to deploy phages to assist in determining whether DNA rearrangements might be responsible for what’s termed “heritable commitment” of cells during the development of multicellular organisms. In the course of development, Smith explains, different cells become committed to different fates in the developing organism — fates that are generally inherited by the cell’s progeny as it divides.
“DNA rearrangements were known to occur regularly in antibody-producing cells,” Smith says. “That’s how each clone of antibody-producing cells becomes committed to making a single antibody. The reason the body can make millions of antibodies is because there are millions of different clones, each committed to making a single antibody. So, the idea was that maybe this was the general way that cells become heritably committed to particular developmental fates, that small rearrangements in their DNA were responsible. I was trying to detect these rearrangements directly.”
Smith says the project went nowhere. The theory was wrong, and his work shed little light as to why. But it did have one major, if unintended, consequence: It piqued Smith’s enthusiasm for phages. “Filamentous phage turned out to be a really good DNA cloning vector,” Smith says. “It had some terrific advantages.”
Smith made use of those advantages in his next project. That work involved exploring the promise and perils of recombinant DNA, a process that shuffles pieces of genetic code among different species.
Many researchers feared such manipulations could open the door to creating powerful, treatment-resistant pathogens. “At that time,” Smith says, “people were really worried about recombinant DNA. There was a big international conference devoted solely to the question of, ‘How can we deal with the possible biological threat of cloned DNAs getting out into the environment and wreaking havoc?’ ”
That conference and subsequent concerns led to a sheaf of federal regulations. One required that all DNA cloning vectors had to be biologically contained. Researchers could clone promising vectors, but to do so they had to demonstrate that the vector could not reproduce organically in the environment. Smith and his team spent months using filamentous phages as a tool to design such a vector.
“It worked beautifully — just in time for all of these DNA guidelines to disappear: No one thought they were needed anymore,” Smith says with a laugh. Not needed, at least, as contained vectors. But by now Smith was convinced, like the generations of phage explorers who came before him, that bacteriophages were both useful and important. Perhaps even worth building a career on.
In the mid-1980s, Smith’s wife, Marjorie, was advancing her own professional life. Sable, a recently retired reproductive health expert and professor of social work at MU, was pursuing a doctorate in public health at the University of North Carolina at Chapel Hill. Smith knew that Bob Webster, a respected biological scientist with his own interest in filamentous phage, was conducting research at nearby Duke. Smith reached out to Webster: Could he perhaps do a research sabbatical in Webster’s lab?
Webster said yes, and Smith soon found himself digging deep into the phages’ coat proteins, those tiny components of their molecular shell. The chief attraction for him involved one particular piece of genetic material located at the tip of the long, thin phage particle, a protein partly buried and partly poking out like an infection-seeking beak. Previous research had determined that the structure of this exposed beak-like gene could be fairly easily manipulated.
“It was flexible,” Smith says. “It seemed very likely that you’d be able to add new protein parts to that protein by fusing two genes. This, you know, was the era of the recombinant DNA revolution. In recombinant DNA research, you take all or part of gene B and all or part of gene A and fuse them together. They now encode a ‘fusion protein’ that has parts of both. It would be just like that, only protein B here wasn’t a stand-alone protein but part of the phage particle.” Smith called these hypothetical structures “fusion phages.”
As his time in Webster’s lab was winding down, Smith turned to Paul Modrich, a Duke biochemist who was to win his own Nobel Prize in 2015. Researchers in Modrich’s lab, which was conveniently located right down the hall, were studying a protein called EcoR1, and had large preparations of the protein, an antibody that bound the protein and the gene for the protein. Smith asked to use these to test his fusion-phage idea. Modrich said he'd be happy to share all three.
Back at his own bench, Smith cut out a piece of the EcoR1 gene and inserted it into the phage’s coat-protein gene. If all went well, he reasoned, new generations of the modified phage would display a piece of the EcoR1 protein on their surfaces, each extended out at the tip.
And so it happened. At this point, the modified phages were still able to infect bacteria. But when Smith introduced the EcoR1 antibody into the mix, this changed, presumably because the antibody had bound to the protein on the surface, preventing infection.
“This was the first indication,” Smith says, “that the phage display concept seemed to be feasible — that it was possible to display foreign proteins, or pieces of proteins, on the surface of phage.”
With just a few days remaining before returning to Mizzou, Smith excitedly told Sable he finally had the result he was looking for.
The sabbatical work was just a start but a meaningful one. Smith now knew phages could be engineered to display a particular peptide. And that these peptides could be used to link a foreign gene’s DNA to the particular proteins he was looking for. So, he wondered, might phages one day usher in an entirely new, highly efficient tool for building vast “libraries” of cloned genes — genes from which new genetic discoveries could be made? Maybe, Smith thought.
Back in his Tucker Hall lab, Smith retooled his research focus. The new agenda would be phage-first. And Steve Parmley, a new graduate student, would be a key part of it.
Now an executive at a San Diego-based biotechnology firm, Parmley, PhD ’88, was just 23 when he joined Smith’s lab. As an undergraduate at the University of Kansas, Parmley had worked with biotechnologists exploring how antibodies might be engineered to attack cancer cells. When the native of St. Louis decided to return to Missouri to do similar work as a doctoral student, Mizzou was an obvious choice. So was George Smith.
Smith and his young collaborator began brainstorming ways to take his invention to the next level. There were a lot of long lunches, Parmley recalls, with the two trading ideas via “back-of-the-envelope cartoons about how to modify the phage as a vector.”
The work at Duke, Parmley continues, relied in part on serendipity. EcoR1 came already equipped with a usable “site” for making genetic fusions and displaying them on its coat protein. Its weakness was its effect on the modified phage; it just wasn’t possible to use it to create vectors in sufficiently large numbers. “My job,” says Parmley, “was to see if we could move that site to somewhere else in the phage coat protein, a place where it would be less detrimental to the viability of the phage.”
Parmley was eventually able to move the site farther away from parts of the phage protein that would inhibit its function. He credits Smith’s “deep knowledge of phage physiology” for making the work possible. At the same time, the two of them laid the groundwork for what came to be known as “affinity selection,” a second core element of phage-display technology.
It worked by using a procedure then common among immunologists. First, Parmley anchored a molecular “bait” directly to a plastic petri dish, in this case an antibody against a protein called beta-galactosidase, which, like Modrich’s EcoRI, served as a convenient model. Parmley then poured a phage-filled solution into the dish. A tiny fraction of the phages were fusion phages displaying parts of the beta-galactosidase protein; the others were wild-type phages that didn’t display any foreign protein. In the dish, the fusion phages were bound by the bait and were captured on the surface of the dish. The wild-type phages weren’t bound and could be washed away.

Smith introduced a gene into the gene for a protein in the phage’s capsule. The phage DNA was then inserted into bacteria that produced phages. The peptide produced from the introduced gene ended up as part of the capsule protein on the surface of the phage. Smith was able to fish out the phage using an antibody designed to attach to the peptide. As a bonus, he got the gene for the peptide. Illustration by Bryan Christie Design.
“George had shown in his initial [1985] Science paper that he could select fusion phages out of a background of wild-type phages with a frequency of about one in a thousand. But we knew that if we were looking for really rare recombinant phage in a large library we’d need to be better than that,” Parmley says. “But because phages are really sticky, some of them would just stick to the surface of the dish nonspecifically. And we couldn’t increase what we were calling the enrichment, or the frequency in which you could recover the fusion phages compared to the wild-type phages.”
The next hurdle involved designing a way to up the level of enrichment so that they could locate more interesting phages out of larger phage libraries — not one desirable phage out of 10,000 but out of a million, a billion, or even, as Smith jokingly likes to put it, a gazillion. They did this by exploiting phages’ remarkable reproductive prowess.
“Because the phages can replicate to huge numbers by infecting fresh bacteria,” Parmley says, “you can reproduce the phages that you’ve recovered from a single round of selection in the petri dish. So, if you grew them back up again and repeated the process with the bait a second time, a third time, each round you got the enrichment of one in a thousand but multiplying that three times you could find something that is one in a billion, one in a gazillion.”
“There are other ways of doing it now,” continues Parmley. “But this was the first one. No one today could imagine trying to screen for genes in the old, laborious ways: There was strict limit to what you can look for. But with phage display, it’s almost unlimited.”
That unlimited potential has since been acknowledged by thousands of researchers around the world, Smith’s Nobel co-laureate, Sir Greg Winter, among them. Winter says he initially thought phage display was a fascinating discovery, though he was skeptical it would amount to much. But one of Winter’s lab assistants, Phil Jennings, was an enthusiastic early adopter, and soon it became a crucial part of Winter’s research program. “It made all the difference,” he says.
Before phage display, Winter says, “we were screening antibody-expression libraries made by secretion from bacteria. We realized that this would not be sufficiently powerful for the very large antibody libraries [we] needed to isolate human antibodies against human self-antigens — as required for treatment of cancer or autoimmune disease.”
Eventually, Winter adds, phage display became a workhorse he and his team used to create “highly diverse and very large human antibody libraries, both from the blood of human donors and from gene segment building blocks.” Such massive libraries, he says, helped distinguish between antibodies that might effectively bind to different therapeutic targets.
Such breakthroughs were crucial to further developments that led to the outcomes noted in the Nobel citation: successful treatments for rheumatoid arthritis, psoriasis and inflammatory bowel diseases. Looking forward, the citation reads, new phage-enabled discoveries are poised to “promote a greener chemicals industry, produce new materials, manufacture sustainable biofuels, mitigate disease and save lives.”
For his part, Smith has retired from his science career. In Sweden, however, he was happy to help the Royal Academy promote phage affection. His schedule, he says, was packed with appearances, not only the official Nobel lecture but also seminars at Swedish universities, appearances at schools, interviews with media, and other official and semiofficial duties. “I got very little sleep, maybe three hours at night. But I was running on adrenalin.”
While onstage at the medal ceremony with his fellow laureates and the Swedish king, Smith’s chief thought was: “Don’t screw it up! Bow the right way! Turn the right way before you bow!” Later, at the official banquet — a lavishly catered dinner that Smith and Sable were able to share with Parmley and several other MU laboratory colleagues — he had one final brush with celebrity.
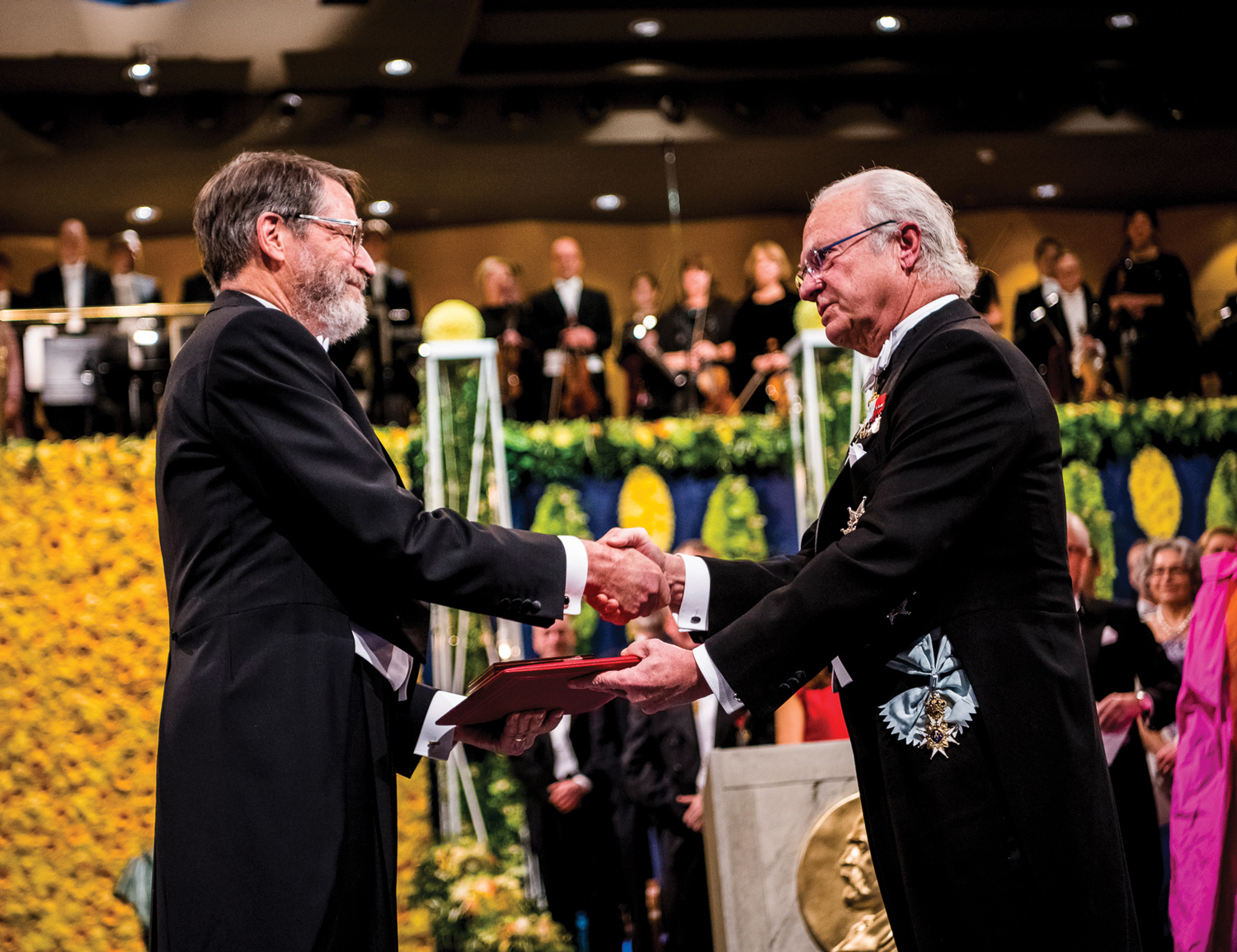
King Carl XVI Gustaf of Sweden handed Smith his Nobel Prize medal and diploma at a ceremony in Stockholm Dec. 10, 2018, the 121st anniversary of Alfred Nobel’s death. View Smith’s Nobel lecture at tinyurl.com/smithnobel. Photo by Alexander Mahmoud.
“At the banquet, each of us was paired with someone really prominent in Swedish society. I was paired with Crown Princess Victoria,” Smith says in a tone of wonder and bemusement. “She was wearing this elaborate gown that her mother, the queen, had worn to the ceremony 20 years earlier. The paparazzi were all over her. So I actually made it into People magazine!
Of course, it was all about her — I’m not even mentioned,” Smith adds with a laugh. “But I’m there.”
To read more MIZZOU magazine stories online, visit mizzou.com.